This is saikrishna !!!!!!!!!!!!!!!
thank you for visiting this website !!!!!!!!!
Light Waves and Color - Lesson 2 - Color and Vision
The Electromagnetic and Visible Spectra:
thank you for visiting this website !!!!!!!!!
Light Waves and Color - Lesson 2 - Color and Vision
The Electromagnetic and Visible Spectra:
Electromagnetic waves exist with an enormous range of frequencies. This continuous range of frequencies is known as the electromagnetic spectrum. The entire range of the spectrum is often broken into specific regions. The subdividing of the entire spectrum into smaller spectra is done mostly on the basis of how each region of electromagnetic waves interacts with matter. The diagram below depicts the electromagnetic spectrum and its various regions. The longer wavelength, lower frequency regions are located on the far left of the spectrum and the shorter wavelength, higher frequency regions are on the far right. Two very narrow regions within the spectrum are the visible light region and the X-ray region. You are undoubtedly familiar with some of the other regions of the electromagnetic spectrum.
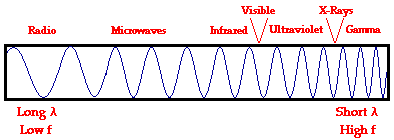
Visible Light Spectrum
The focus of Lesson 2 will be upon the visible light region - the very narrow band of wavelengths located to the right of the infrared region and to the left of the ultraviolet region. Though electromagnetic waves exist in a vast range of wavelengths, our eyes are sensitive to only a very narrow band. Since this narrow band of wavelengths is the means by which humans see, we refer to it as the visible light spectrum. Normally when we use the term "light," we are referring to a type of electromagnetic wave that stimulates the retina of our eyes. In this sense, we are referring to visible light, a small spectrum from the enormous range of frequencies of electromagnetic radiation. This visible light region consists of a spectrum of wavelengths that range from approximately 700 nanometers (abbreviated nm) to approximately 400 nm. Expressed in more familiar units, the range of wavelengths extends from 7 x 10-7 meter to 4 x 10-7 meter. This narrow band of visible light is affectionately known as ROYGBIV.Each individual wavelength within the spectrum of visible light wavelengths is representative of a particular color. That is, when light of that particular wavelength strikes the retina of our eye, we perceive
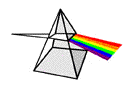
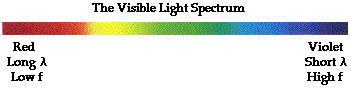
When all the wavelengths of the visible light spectrum strike your eye at the same time, white is perceived. The sensation of white is not the result of a single color of light. Rather, the sensation of white is the result of a mixture of two or more colors of light. Thus, visible light - the mix of ROYGBIV - is sometimes referred to as white light. Technically speaking, white is not a color at all - at least not in the sense that there is a light wave with a wavelength that is characteristic of white. Rather, white is the combination of all the colors of the visible light spectrum. If all the wavelengths of the visible light spectrum give the appearance of white, then none of the wavelengths would lead to the appearance of black. Once more, black is not actually a color. Technically speaking, black is merely the absence of the wavelengths of the visible light spectrum. So when you are in a room with no lights and everything around you appears black, it means that there are no wavelengths of visible light striking your eye as you sight at the surroundings.
Investigate!
Match a Wavelength of Light to a Color | ||
---|---|---|
Check Your Understanding
a. can travel through materials and mechanical waves cannot
b. come in a range of frequencies and mechanical waves exist with only certain frequencies
c. can travel through a region void of matter and mechanical waves cannot
d. electromagnetic waves cannot transport energy and mechanical waves can transport energy
e. electromagnetic waves have an infinite speed and mechanical waves have a finite speed
2. Consider the electromagnetic spectrum as you answer these three questions.
a. Which region of the electromagnetic spectrum has the highest frequency?
b. Which region of the electromagnetic spectrum has the longest wavelength?
c. Which region of the electromagnetic spectrum will travel with the fastest speed?
a. Which color of the visible light spectrum has the greatest frequency?ANSWERS:
b. Which color of the visible light spectrum has the greatest wavelength?
1.Answer: C
Electromagnetic waves are able to travel through a vacuum - a region void of matter. Mechanical waves require a medium in order to propagate from one location to another.
2.a. The gamma radiation region have the highest frequency.
b. The radio wave region has the longest wavelength.
c. All regions have the same speed. The speed of a wave is not dependent upon its frequency and wavelength but rather upon the properties of the medium through which it travels.
3.a. Violet waves have the highest frequencies.
b. Red waves have the longest wavelengths.
Visible Light and the Eye's Response
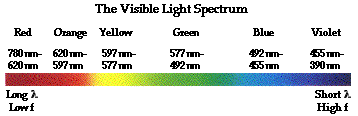
Color Cones
Color can be thought of as a psychological and physiological response to light waves of a specific frequency or set of frequencies impinging upon the eye. An understanding of the human response to color demands that one understand the biology of the eye. Light that enters the eye through the pupil ultimately strikes the inside surface of the eye known as the retina. The retina is lined with a variety of light sensing cells known as rods and cones. While the rods on the retina are sensitive to the intensity of light, they cannot distinguish between lights of different wavelengths. On the other hand, the cones are the color-sensing cells of the retina. When light of a given wavelength enters the eye and strikes the cones of the retina, a chemical reaction is activated that results in an electrical impulse being sent along nerves to the brain. It is believed that there are three kinds of cones, each sensitive to its own range of wavelengths within the visible light spectrum. These three kinds of cones are referred to as red cones, green cones, and blue cones because of their respective sensitivity to the wavelengths of light that are associated with red, green and blue. Since the red cone is sensitive to a range of wavelengths, it is not only activated by wavelengths of red light, but also (to a lesser extent) by wavelengths of orange light, yellow light and even green light. In the same manner, the green cone is most sensitive to wavelengths of light associated with the color green. Yet the green cone can also be activated by wavelengths of light associated with the colors yellow and blue. The graphic below is a sensitivity curve that depicts the range of wavelengths and the sensitivity level for the three kinds of cones.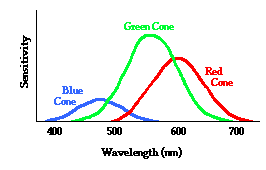
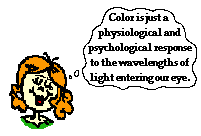
Now suppose that light in the yellow range of wavelengths (approximately 577 nm to 597 nm) enters the eye and strikes the retina. Light with these wavelengths would activate both the green and the red cones of the retina. Upon striking the retina, the physiological occurs: electrical messages are sent by both the red and the green cones to the brain. Once received by the brain, the psychological occurs: the brain recognizes that the light has activated both the red and the green cones and somehow interprets this to mean that the object is yellow. In this sense, the yellow appearance of objects is simply the result of yellow light from the object entering our eye and stimulating the red and the green cones simultaneously.
If the appearance of yellow is perceived of an object when it activates the red and the green cones simultaneously, then what appearance would result if two overlapping red and green spotlights entered our eye? Using the same three-cone theory, we could make some predictions of the result. Red light entering our eye would mostly activate the red color cone; and green light entering our eye would mostly activate the green color cone. Each cone would send their usual electrical messages to the brain. If the brain has been psychologically trained to interpret these two signals to mean "yellow", then the brain would perceive the overlapping red and green spotlights to appear as yellow. To the eye-brain system, there is no difference in the physiological and psychological response to yellow light and a mixing of red and green light. The brain has no means of distinguishing between the two physical situations.
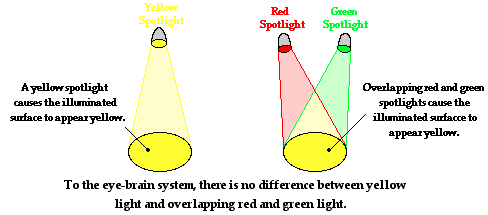
In a technical sense, it is really not appropriate to refer to light as being colored. Light is simply a wave with a specific wavelength or a mixture of wavelengths; it has no color in and of itself. An object that is emitting or reflecting light to our eye appears to have a specific color as the result of the eye-brain response to the
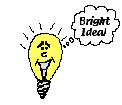
In the next several sections of Lesson 2, we will explore these concepts further by introducing three primary colors of light and generating some simple rules for predicting the color appearance of objects in terms of the three primary colors.
Investigate!
Light Absorption, Reflection, and Transmission
Visible Light Absorption
Atoms and molecules contain electrons. It is often useful to think of these electrons as being attached to the atoms by springs. The electrons and their attached springs have a tendency to vibrate at specific frequencies. Similar to a tuning fork or even a musical instrument, the electrons of atoms have a natural frequency at which they tend to vibrate. When a light wave with that same natural frequency impinges upon an atom, then the electrons of that atom will be set into vibrational motion. (This is merely another example of the resonance principle introduced in Unit 11 of The Physics Classroom Tutorial.) If a light wave of a given frequency strikes a material with electrons having the same vibrational frequencies, then those electrons will absorb the energy of the light wave and transform it into vibrational motion. During its vibration, the electrons interact with neighboring atoms in such a manner as to convert its vibrational energy into thermal energy. Subsequently, the light wave with that given frequency is absorbed by the object, never again to be released in the form of light. So the selective absorption of light by a particular material occurs because the selected frequency of the light wave matches the frequency at which electrons in the atoms of that material vibrate. Since different atoms and molecules have different natural frequencies of vibration, they will selectively absorb different frequencies of visible light.Visible Light Reflection and Transmission
Reflection and transmission of light waves occur because the frequencies of the light waves do not match the natural frequencies of vibration of the objects. When light waves of these frequencies strike an object, the electrons in the atoms of the object begin vibrating. But instead of vibrating in resonance at a large amplitude, the electrons vibrate for brief periods of time with small amplitudes of vibration; then the energy is reemitted as a light wave. If the object is transparent, then the vibrations of the electrons are passed on to neighboring atoms through the bulk of the material and reemitted on the opposite side of the object. Such frequencies of light waves are said to be transmitted. If the object is opaque, then the vibrations of the electrons are not passed from atom to atom through the bulk of the material. Rather the electrons of atoms on the material's surface vibrate for short periods of time and then reemit the energy as a reflected light wave. Such frequencies of light are said to be reflected.Where Does Color Come From?
The color of the objects that we see is largely due to the way those objects interact with light and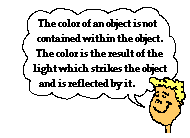
Consider the two diagrams below. The diagrams depict a sheet of paper being illuminated with white light (ROYGBIV). The papers are impregnated with a chemical capable of absorbing one or more of the colors of white light. Such chemicals that are capable of selectively absorbing one or more frequency of white light are known as pigments. In Example A, the pigment in the sheet of paper is capable of absorbing red, orange, yellow, blue, indigo and violet. In Example B, the pigment in the sheet of paper is capable of absorbing orange, yellow, green, blue, indigo and violet. In each case, whatever color is not absorbed is reflected.

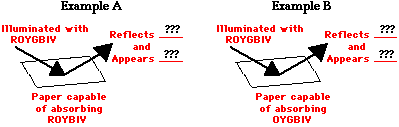

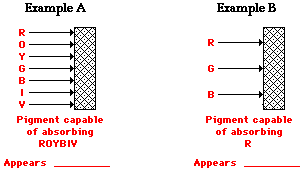
Check Your Understanding
2. What color does a red shirt appear when the room lights are turned off and the room is entirely dark? ____________ What about a blue shirt? ____________ ... a green shirt? ____________
3. The diagrams depict a sheet of paper being illuminated with white light (ROYGBIV). The papers are impregnated with a chemical capable of absorbing one or more of the colors of white light. In each case, determine which color(s) of light are reflected by the paper and what color the paper will appear to an observer.
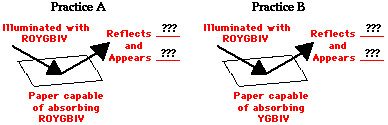
4. The appearance of a transparent object is dependent upon which color(s) of light is/are incident upon the object and which color(s) of light is/are transmitted through the object. Express your understanding of this principle by determining which color(s) of light will be transmitted and the color that the paper will appear to an observer.
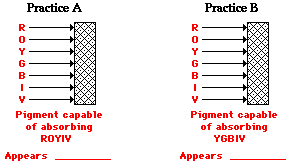
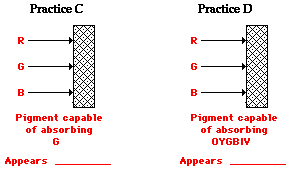
Quiz answers:
1.Example A: Green will be reflected and so the paper appears green to an observer.
Example B: Red will be reflected and so the paper appears red to an observer.
CHECKING YOUR UNDERSTANDING ANSWERS:
1.Answer: Not accurate
This view presumes that the appearance of an object is independent of
the colors of light which illuminate the object. We observe that the
same object appears different colors when viewed under different light.
So the secret to an object's appearance is not strictly due to its
ability to produce a color. In fact the object's only role in
determining its appearance is in its ability to absorb certain
wavelengths of light which shine upon it.
2.Answer: Black
When the room lights are turned off (there is no light), any object
present in the room appears black. The color appearance of an object
depends upon the light which that objects reflects to the observer's
eye. Without any incident light, there can be no reflected light. Such
an object appears black - the absence of light.
3.Practice A: No light will be reflected; it is all absorbed. Thus, the paper would appear black to an observer.
Practice B: Red and orange will be reflected and so the paper appears reddish-orange to an observer.
4. a)Practice A: Green and blue light will be transmitted and so the object would appear greenish-blue to an observer.
Practice B: Red and orange light will be transmitted and so the object would appear reddish-orange to an observer.
b)Practice C: Red and blue light will be transmitted and so the object would appear reddish-blue to an observer.
Practice D: Only red light will be transmitted and so the object would appear red to an observer.
Color Addition
Primary Colors of Light
The subject of color perception can be simplified if we think in terms of primary colors of light. We have already learned that white is not a color at all, but rather the presence of all the frequencies of visible light. When we speak of white light, we are referring to ROYGBIV - the presence of the entire spectrum of visible light. But combining the range of frequencies in the visible light spectrum is not the only means of producing white light. White light can also be produced by combining only three distinct frequencies of light, provided that they are widely separated on the visible light spectrum. Any three colors (or frequencies) of light that produce white light when combined with the correct intensity are called primary colors of light. There are a variety of sets of primary colors. The most common set of primary colors is red (R), green (G) and blue (B). When red, green and blue light are mixed or added together with the proper intensity, white (W) light is obtained. This is often represented by the equation below:The addition of the primary colors of light can be demonstrated using a light box. The light box illuminates a screen with the three primary colors - red (R), green (G) and blue (B). The lights are often the shape of circles. The result of adding two primary colors of light is easily seen by viewing the overlap of the two or more circles of primary light. The different combinations of colors produced by red, green and blue are shown in the graphic below. (CAUTION: Because of the way that different monitors and different web browsers render the colors on the computer monitor, there may be slight variations from the intended colors.)
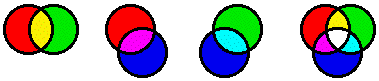
Color Addition Rules
These demonstrations with the color box illustrate that red light and green light add together to produce yellow (Y) light. Red light and blue light add together to produce magenta (M) light. Green light and blue light add together to produce cyan (C) light. And finally, red light and green light and blue light add together to produce white light. This is sometimes demonstrated by the following color equations and graphic:R + G = Y |
![]() |
Yellow (Y), magenta (M) and cyan (C) are sometimes referred to as secondary colors of light since they can be produced by the addition of equal intensities of two primary colors of light. The addition of these three primary colors of light with varying degrees of intensity will result in the countless other colors that we are familiar (or unfamiliar) with.
Investigate!
Color Addition | ||
---|---|---|
Complementary Colors of Light
Any two colors of light that when mixed together in equal intensities produce white are said to be complementary colors
of each other. The complementary color of red light is cyan light. This
is reasonable since cyan light is equivalent to a combination of blue
and green light; and blue and green light when added to red light will
produce white light. Thus, red light and cyan light (which is equivalent
to blue + green light) represent a pair of complementary colors of
light; they add together to produce white light. This is illustrated in
the equation below:Complementary Colors of Light |
![]() |
The production of various colors of light by the mixing of the three primary colors of light is known as color addition. The color addition principles discussed on this page can be used to make predictions of the colors that would result when different colored lights are mixed. In the next part of Lesson 2, we will learn how to use the principles of color addition to determine why different objects look specific colors when illuminated with various colors of light.
Check Your Understanding
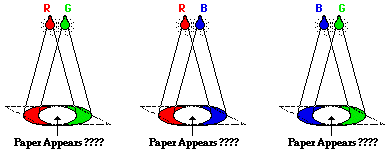
2. Suppose that light from a magenta spotlight and light from a yellow spotlight are mixed together, will white light be produced? Explain.
ANSWERS:
1.R + G ---> Yellow
R + B ---> Magenta
B + G ---> Cyan
2.Answer: No
The magenta spotlight can be thought of as a combination of red and blue light in equal intensities and the yellow spotlight is equivalent to a combination of red and green light in equal intensities. Observe the double abundance of red. Combining the light from the magenta and yellow spotlights will produce a whitish-red color - that is, pink.
Color Subtraction
We have already learned that materials contain atoms that are capable of selectively absorbing one or more frequencies of light. Consider a shirt made of a material that is capable of absorbing blue light. Such a material will absorb blue light (if blue light shines upon it) and reflect the other frequencies of the visible spectrum. What appearance will such a shirt have if illuminated with white light and how can we account for its appearance? To answer this question (and any other similar question), we will rely on our understanding of the three primary colors of light (red, green and blue) and the three secondary colors of light (magenta, yellow and cyan).
The Process of Color Subtraction
To begin, consider white light to consist of the three primary colors of
light - red, green and blue. If white light is shining on a shirt, then
red, green and blue light is shining on the shirt. If the shirt absorbs
blue light, then only red and green light will be reflected from the
shirt. So while red, green and blue light shine upon the shirt, only red
and green light will reflect from it. Red and green light striking your
eye always gives the appearance of yellow; for this reason, the shirt
will appear yellow. This discussion illustrates the process of color subtraction.
In this process, the ultimate color appearance of an object is
determined by beginning with a single color or mixture of colors and
identifying which color or colors of light are subtracted from the
original set. The process is depicted visually by diagram at the right.
Furthermore, the process is depicted in terms of an equation in the
space below.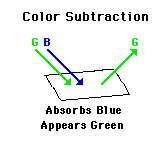
C - B = (G + B) - B = G
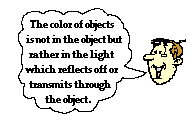

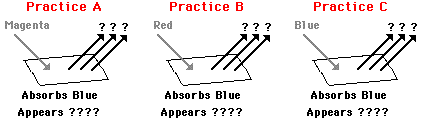
Practice A: Magenta light is a mixture of red light and blue light in equal intensities. Blue light must be subtracted since it is absorbed. When subtracting blue light from red and blue light, the red remains. The shirt appears red.
Practice B: Red light is a primary color. Blue light would have to be subtracted if present. Since it is not present, there is no need to worry about it. Red light is reflected and the shirt appears red.
Practice C: Blue light is a primary color. Blue light must be subtracted since it is absorbed. There is no other color left to reflect to our eyes. The shirt appears black since black is the absence of reflected light.
(R + B) - B = R
Practice B: Red light is a primary color. Blue light would have to be subtracted if present. Since it is not present, there is no need to worry about it. Red light is reflected and the shirt appears red.
Practice C: Blue light is a primary color. Blue light must be subtracted since it is absorbed. There is no other color left to reflect to our eyes. The shirt appears black since black is the absence of reflected light.
Complementary Colors and Color Subtraction
In the above examples, the paper absorbed blue light. Paper that absorbs blue light is permeated by a pigment known as a yellow pigment. While most pigments absorb more than a single frequency (and are known as compound pigments), it becomes convenient for our discussion to keep it simple by assuming that a yellow pigment absorbs a single frequency. A pigment that absorbs a single frequency is known as a pure pigment. The following rule will assist in understanding what colors of light are absorbed by which pigments.Thus, pure blue pigments absorb yellow light (which can be thought of as a combination of red and green light). Pure yellow pigments absorb blue light. Pure green pigments absorb magenta light (which can be thought of as a combination of red and blue light). Pure magenta pigments absorb green light. Pure red pigments absorb cyan light (which can be thought of as a combination of blue and green light). And finally, pure cyan pigments absorb red light.
Pigments absorb light. Pure pigments absorb a single frequency or color of light. The color of light absorbed by a pigment is merely the complementary color of that pigment.
Now lets combine the process of color subtraction with an understanding of complementary colors to determine the color appearance of various sheets of paper when illuminated by various lights. We will investigate three examples.
Example 1 |
Example 2 |
Example 3 |
Flickr Physics Photo
Filters and Color Subtraction
The above discussion applies to the appearance of opaque materials. The distinction between opaque and transparent materials was made earlier in this lesson. Opaque materials selectively absorb one or more frequencies of light and reflect what is not absorbed. In contrast to opaque materials, transparent materials selectively absorb one or more frequencies of light and transmit what is not absorbed. Like opaque materials, transparent materials are permeated by pigments that contain atoms that are capable of absorbing light with a single frequency or even a range of frequencies. Knowing the color(s) of the incident light and the color of light absorbed by the pigment or filter, the process of color subtraction can be applied to determine the color appearance of a transparent material. We will consider three examples in the space below; the examples are visually depicted in the diagrams below.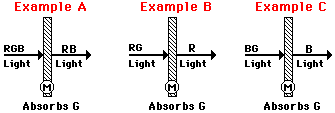
The reasoning modeled in the above three examples can be used in any situation, regardless of the color of the incident light and the color of the filter. As you approach such problems, whether they involve transparent or opaque materials, be sure to think in terms of primary colors of light and to use the logical reasoning steps. Avoid memorizing and avoid shortcuts. If a filter is capable of absorbing a color of light that is not present in the mixture of incident light, then merely disregard that color. Since that color of light is not incident upon the object, it cannot contribute to the color appearance of the object.
Primary Colors of Paint
A trip to the local newspaper or film developing company will reveal these same principles of color subtraction at work. The three primary colors of paint used by an artist, color printer or film developer are cyan (C), magenta (M), and yellow (Y). Artists, printers, and film developers do not deal directly with light; rather, they must apply paints or dyes to a white sheet of paper. These paints and dyes must be capable of absorbing the appropriate components of white light in order to produce the desired affect. Most artists start with a white canvas and apply paints. These paints have to subtract colors so that you might see the desired image. An artist can create any color by using varying amounts of these three primary colors of paint.Each primary color of paint absorbs one primary color of light. The color absorbed by a primary color of paint is the complementary color of that paint. The three colors that are primary to an artist (magenta, cyan, and yellow) subtract red, green, and blue individually from an otherwise white sheet of paper. Thus,
Cyan paints absorb red light.
Yellow paints absorb blue light.
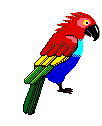
To produce a green tail, paints must be applied to the tail region in order to absorb red and blue light and leave green to be reflected. Thus, the green tail must be painted using yellow paint (to absorb the blue) and cyan paint (to absorb the red).
To produce a blue lower body, paints must be applied to the lower body region in order to absorb red and green light, leaving blue light to be reflected. Thus, the blue lower body must be painted using magenta paint (to absorb the green) and cyan paint (to absorb the red).
To produce a red head, paints must be applied to the head region in order to absorb blue and green light, leaving red light to be reflected. Thus, the red head must be painted using magenta paint (to absorb the green) and yellow paint (to absorb the blue).
To produce a cyan upper body, paints must be applied to the upper body region in order to absorb red, leaving green and blue light to be reflected. If green and blue light are reflected from the upper body region, it will appear cyan (recall that blue and green light combine to form cyan light). Thus, the cyan upper body must be painted using merely cyan paint (to absorb the red).
To produce a magenta eye patch, paints must be applied to the eye patch region in order to absorb green, leaving red and blue light to be reflected. If red and blue light is reflected from the eye patch region, it will appear magenta (recall that blue and red light combine to form magenta light). Thus, the magenta eye patch must be painted using merely magenta paint (to absorb the green).
To produce a yellow eye and middle feathers, paints must be applied to the eye and middle feather regions in order to absorb blue, leaving red and green light to be reflected. If red and green light is reflected from the eye and middle feather regions, it will appear yellow (recall that red and green light combine to form yellow light). Thus, the yellow eye and middle feathers must be painted using merely yellow paint (to absorb the blue).
This information is summarized in the graphic below.
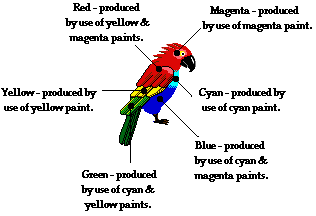
The process of color subtraction is a useful means of predicting the ultimate color appearance of an object if the color of the incident light and the pigments are known. By using the complementary color scheme, the colors of light that will be absorbed by a given material can be determined. These colors are subtracted from the incident light colors (if present) and the colors of reflected light (or transmitted light) can be determined. Then the color appearance of the object can be predicted.
Investigate!
Blue Skies and Red Sunsets
- Why are the skies blue?
- Why are the sunsets red?
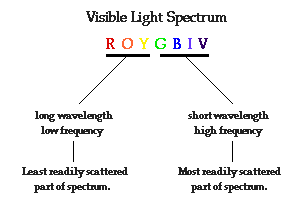
Why are the skies blue?
The interaction of sunlight with matter can result in one of three wave behaviors: absorption, transmission, and reflection.
The atmosphere is a gaseous sea that contains a variety of types of
particles; the two most common types of matter present in the atmosphere
are gaseous nitrogen and oxygen. These particles are most effective in
scattering the higher frequency and shorter wavelength portions of the
visible light spectrum. This scattering process involves the absorption
of a light wave by an atom followed by reemission of a light wave in a
variety of directions. The amount of multidirectional scattering that
occurs is dependent upon the frequency of the light. (In fact, it varies
according to f4.) Atmospheric nitrogen and oxygen scatter
violet light most easily, followed by blue light, green light, etc. So
as white light (ROYGBIV) from the sun passes through our atmosphere, the
high frequencies (BIV) become scattered by atmospheric particles while
the lower frequencies (ROY) are most likely to pass through the
atmosphere without a significant alteration in their direction. This
scattering of the higher frequencies of light illuminates the skies with
light on the BIV end of the visible spectrum. Compared to blue light,
violet light is most easily scattered by atmospheric particles. However,
our eyes are more sensitive to light with blue frequencies. Thus, we
view the skies as being blue in color.Why are sunsets red?
Meanwhile, the light that is not scattered is able to pass through our atmosphere and reach our eyes in a rather non-interrupted path. The lower frequencies of sunlight (ROY) tend to reach our eyes as we sight directly at the sun during midday. While sunlight consists of the entire range of frequencies of visible light, not all frequencies are equally intense. In fact, sunlight tends to be most rich with yellow light frequencies. For these reasons, the sun appears yellow during midday due to the direct passage of dominant amounts of yellow frequencies through our atmosphere and to our eyes.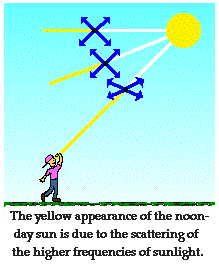
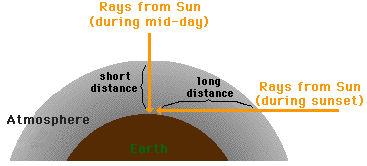
The Wonders of Physics
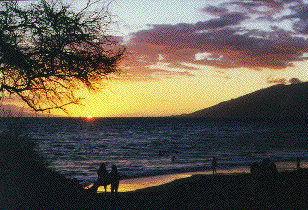
Photograph of Maui sunset by Becky Henderson
No comments:
Post a Comment